![[NAAPO Logo]](../../Images/NAAPOsm.jpg)
North American AstroPhysical Observatory (NAAPO)
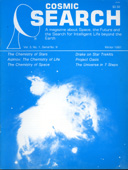
Cosmic Search: Issue 9 (Volume 3 Number 1; Winter 1981) [All Articles & Miscellaneous Items]
|
Webpage Table of Contents (Bookmarks)
(Internal links to items in this webpage)
[Note. Use Back button (or <Alt>+<Left Arrow>) to get back to this Table of Contents after you have clicked on a link and viewed the article.]
Codes Used Below:
P: Starting page of article in magazine; A: Author(s); T: Title of article
SETI and the Spectral Classification of Stars
By: J.D.R. Bahng
It is generally agreed that the most reasonable SETI* (*SETI: Search for Extra-Terrestrial Intelligence.) strategy available today involves the detection and
identification of intelligent signals in the microwave region of the electromagnetic spectrum — the so-called water hole.** (**See COSMIC SEARCH, Serial no. 1, Jan. 1979, page 35.) To do this we need at least one large radio telescope equipped with a very sensitive detector and a smart computer analyzer. In two NASA-sponsored summer projects (Project Cyclops in 1971 and Project Oasis in 1979) studies were made to define parameters of these systems.
The results show that we now have means and capabilities to start a serious SETI program.
There is a possibility that in the near future we may have at our disposal a very sophisticated optical system in space capable of registering the actual images of planets around distant stars.
In either case, the matter of economy and efficiency dictates that the search list be limited to the most likely stars — the "good" stars. There are some 200 billion stars in our Galaxy. Even if we confine ourselves to a space within 1000 light-years from the Sun, a more likely range limit for our technology within a foreseeable future, there are 10 million stars. It is estimated that only about 20 percent of all stars are likely to have planets.*** (***See COSMIC SEARCH, Serial no. 1, Jan. 1979, page 34.) Thus, we must take a census and select some 2 million out of these 10 million stars. Our concern then is; what are the most important characteristics common to good stars?
Of all the stars in the Universe, we know of one that is definitely a good star: our Sun. It is "good" because it has a planet, the Earth, which has life. Because of its proximity we know quite a bit about the Sun. Among other things, we know its mass, diameter, luminosity, and surface temperature. If we find a remote star whose properties are identical or very similar to our Sun, we can be quite certain that it would be a good star. For most stars, however, it is not possible to determine all these characteristics directly. Even the indirect determination is difficult and time consuming in most instances.
Fortunately for us, it is not necessary to determine all there is to know about each star. It is true that every star is different from any other star in some respect, but there are sufficient similarities amongst stars that millions and billions of stars can be classified into a very small number of classes or types by rather a limited set of parameters. The most useful and one of the easiest to obtain is the spectral type.* (*When the light of a star is passed through a prism it may be spread into a spectrum or rainbow of colors, each color representing a different wavelength. From such stellar spectra stars can be classified. — Ed.)
How Astronomers Classify Stars and What This Means to SETI
Near the end of the 19th century photographic studies of the spectra of stars were initiated.* (*When the light of a star is passed through a prism it may be spread into a spectrum or rainbow of colors, each color representing a different wavelength. From such stellar spectra stars can be classified. — Ed.) Soon hundreds of thousands of stellar spectra were collected. A classification scheme based on the appearance of stellar spectra in the blue-violet to green region, (the region for which the ordinary photographic emulsion is most sensitive), was undertaken by Annie J. Cannon and her collaborators at Harvard College Observatory.
Initially, the letter A was assigned to those stars whose spectra appeared the simplest, and successive letters of alphabet were used for more and more complex spectra. As the scheme evolved, some of the types were merged and certain letters were dropped. At the same time, it was realized that the changing features of spectra of stars were almost exclusively due to the temperature in the outer layers of the stars. That is, the spectral classification actually arranges stars in order of their surface temperatures. There is a correlation between the star's surface temperature and its characteristic color; the cool stars appear reddish while the very hot stars appear bluish. By the time Annie Cannon's work was completed and published as the Henry Draper (HD) Catalogue in 1924, nearly 99 percent of over 220,000 stars in this catalogue could be classified into seven principal classes or types. These are, in order of decreasing temperatures: O, B, A, F, G, K, and M. There is a continuous gradation from one type to the next so that each type is subdivided into ten subclasses denoted by numbers 0 through 9. Thus, a star whose spectrum appears to fall half-way between the types B0 and A0 would be classified as B5. In recent years, some improvement and modifications were introduced, but the essential feature of the Harvard Classification scheme is still in use today.
Principal features and temperature range of each spectral type are as follows:
O: Bluish. Lines of ionized helium. Hydrogen lines are quite weak. Temperatures are 30,000 K
(30,000 degrees kelvin) or higher.
B: Blue-white. Lines of neutral helium. Hydrogen lines are more prominent than in O stars. Temperatures are around 20,000 kelvin.
A: White. Very strong lines of hydrogen dominate the appearance of spectra. Temperatures are around 10,000 kelvin.
F: White. Hydrogen lines are weaker than in A stars, but still conspicuous. Lines of ionized calcium and other metals begin to show. Temperatures in the range of 7,000 to 8,000 kelvin.
G: Yellowish. Lines of ionized calcium are the most conspicuous, hydrogen lines are weaker than in F stars. Molecular bands of CH are present. Temperatures in the range of 5,000 to 6,000 kelvin.
K: Orange. Numerous lines of neutral metals predominate. The CH bands as well as CN bands are present. Temperatures are around 4,000 to 5,000 kelvin.
M: Reddish. Strong lines of neutral metals and molecular bands of titanium oxide dominate the entire spectrum. Temperatures are below 3,500 kelvin.
The spectral type of the Sun is G2. As one might expect, there are underlying physical principles that determine the various properties of the stars, so that all the stars belonging to a particular spectral type, say A0, have more or less identical physical parameters with some outstanding exceptions as shown in the Hertzsprung-Russell Diagram (H-R diagram for short). It is named after two astronomers, Enjar Hertzsprung of Denmark and Henry Norris Russell of Princeton University, who
first made such diagrams around 1914 independently of each other.
The total luminosity of a star, that is the total power radiated by the star as a whole, is plotted against its spectrum type. A great majority of the stars fall into a diagonal region on this diagram called the Main Sequence. Along the Main Sequence, going from the blue O stars toward red M stars, we find that both the luminosity and surface temperature decline together. It is also found that the total mass decreases in the same direction. Thus blue O stars are the most massive, most luminous and hottest, while red M stars the least massive, least luminous and coolest. A yellow G star, including our Sun, can be considered as an average star.
Some stars are found to lie above and to the right of the Main Sequence. For these stars, the spectral type is the same but they are intrinsically more luminous than the corresponding Main Sequence stars. These stars are called giants. Since the same spectral type implies the same surface temperature, the giant stars must actually be larger in size to have higher luminosity. In general, these giant stars are 10 to 100 times larger in size than the corresponding Main Sequence stars.
If one compares the spectrum of a Main Sequence G2 star with that of giant G2 star, the overall appearance of the two are almost identical but there are some minor but significant differences. This is called a luminosity effect. Thus, a careful inspection of star's spectrum enables us to distinguish between the Main Sequence and giant stars of the same spectral type.
Beginning in the 1940's, William W. Morgan and Philip C. Keenan, at Yerkes Observatory, University of Chicago, made detailed studies of luminosity effects in the stellar spectra. Morgan and Keenan, who is now at Perkins Observatory, refined the original Harvard system by incorporating five luminosity classes, designated by Roman numerals I through V. Stars of the luminosity class I are the supergiants, the most luminous stars, while those of class V are the Main Sequence (or dwarf)
stars.
Refiners of spectral designations into the M-K luminosity classes.
The new system now in use is called the two-dimensional spectral classification: the spectral type (as in the original Harvard system) followed by Morgan and Keenan's (M-K) luminosity class. In this scheme, the Sun is classified as G2 V (yellow dwarf), while the star Rigel in the constellation Orion is B8 Ia (Blue supergiant) (the class I is further subdivided into a and b with Ia being the more luminous of the most luminous supergiants).
According to the current understanding of stellar evolution, the Main Sequence is where the stars spend most of their life. Giants and supergiants are evolved stars which, having spent their allotted time on the Main Sequence, become more luminous and larger, and are on their way toward eventual extinction. It is beyond the scope of this paper to discuss in detail why some stars are "good" suns while others are not. But briefly, the following picture emerges.
The giants and supergiants are ruled out because of their high luminosity (large power output) and their large physical size; any habitable planets that might have surrounded them initially would have been destroyed. Along the Main Sequence, O to about F5 stars (blue and white stars) are excluded for a couple of reasons. First, they all seem to rotate very fast, indicating that these stars are not likely to harbor any planets. Second, and perhaps more serious is the fact that their Main Sequence life time is much too short, less than 1 billion years, before they evolve into giants or supergiants.
At the other end of the classification, most of the M red dwarf stars exhibit rather violent flare activities, pouring out radiation and charged particles that may destroy the favorable environment for life on planets around these stars. Thus, we are left with G, K, (yellow and orange) and possibly some Main Sequence F stars as the prime candidates for good stars. It is not surprising that our Sun is in the group.
Our task is now clear. We must sift through some 10 million stars within 1000 light-years from the Sun and pick out G and K (yellow and orange) Main Sequence stars by identifying their spectral features. This must be done rapidly, economically and accurately. It is hoped that such an efficient large-scale spectral classification method becomes available very soon.
John D. R. Bahng has been a member of the astronomy faculty of Northwestern University (Evanston, Illinois) since 1962 and the Director of the Dearborn Observatory and the Lindheimer Astronomical Research Center since 1975.
Born in Korea in 1927 he holds a B.S. degree from St. Norbert College (DePere, Wisconsin) and M.S. and Ph.D. degrees from the University of Wisconsin (Madison). Dr. Bahng's special interests include photoelectric spectrophotometry and astronomical instrumentation, areas in which he has had extensive experience.
|
Not as We Know it
The Chemistry of Life
By: Isaac Asimov
Even unpleasant experiences can be inspiring.
For instance, my children once conned me into taking them to a monster-movie they had seen advertised on TV. "It's science fiction," they explained. They don't exactly know what science fiction is, but they have gathered it's something daddy writes, so the argument is considered very powerful.
I tried to explain that it wasn't science fiction by my definition, but although I had logic on my side, they had decibels on theirs.
So I joined a two-block line consisting of every kid for miles around with an occasional grown-up who spent his time miserably pretending he was waiting for a bus and would leave momentarily. It was a typical early spring day in New England — nasty drizzle whipped into needle-spray by a howling east wind — and we inched slowly forward.
Finally, when we were within six feet of the ticket-sellers and I, personally, within six inches of pneumonia, my guardian angel smiled and I had my narrow escape. They hung up the SOLD OUT sign.
I said, with a merry laugh, "Oh, what a dirty shame," and drove my howling indignant children home. Anyway, it got me to thinking about the lack of imagination in movieland's monsters. Their only attributes are their bigness and destructiveness. They include big apes, big octopuses (or is the word "octopodes"?), big eagles, big spiders, big amoebae. In a way, that is all Hollywood needs, I suppose. This alone suffices to drag in huge crowds of vociferous human larvae, for to be big and
destructive is the secret dream of every red-blooded little boy and girl in the world.
What, however, is mere size to the true aficionado? What we want is real variety. When the cautious astronomer speaks of life on other worlds with the qualification "life-as-we-know-it," we become impatient. What about life-not-as-we-know-it?
Well, that's what I want to discuss.
To begin with, we have to decide what life-as-we-know-it, means. Certainly life-as-we-know-it is infinitely various. It flies, runs, leaps, crawls, walks, hops, swims, and just sits. It is green, red, yellow, pink, dead white and vari-colored. It glows and does not glow, eats and does not eat. It is boned, shelled, plated and soft; has limbs, tentacles or no appendages at all; it is hairy, scaly, feathery, leafy, spiny and bare.
If we're going to lump it all as life-as-we-know-it, we'll have to find out something it all has in common. We might say it is all composed of cells, except that this is not so. The virus, an important life form to anyone who has ever had a cold, is not.
So we must strike beyond physiology and reach into chemistry, saying that all life is made up of a directing set of nucleic acid molecules which controls chemical reactions through the agency of proteins working in a watery medium.
There is more, almost infinitely more, to the details of life, but I am trying to strip it to a basic minimum. For life-as-we-know-it, water is the indispensable background against which the drama is played out, and nucleic acids and proteins are the featured players.
Hence any scientist, in evaluating the life possibilities on any particular world, instantly dismisses said world if it lacks water; or if it possesses water outside the liquid range, in the form of ice only or of steam only.
(You might wonder, by the way, why I don't include oxygen as a basic essential. I don't because it isn't. To be sure, it is the substance most characteristically involved in the mechanics by which most life forms evolve energy, but it is not invariably involved. There are tissues in our body that can live temporarily in the absence of molecular oxygen, and there are microorganisms that can live indefinitely in the absence of oxygen. Life on earth almost certainly developed in an oxygen-free atmosphere, and even today there are microorganisms that can live only in the absence of oxygen. No known life form on earth, however, can live in the complete absence of water, or fails to contain both protein and nucleic acid.)
In order to discuss life-not-as-we-know-it, let's change either the background or the feature players. Background first!
Water is an amazing substance with a whole set of unusual properties which are ideal for life-as-we-know-it. So well fitted for life is it, in fact, that some people have seen in the nature of water a sure sign of Divine providence. This, however, is a false argument, since life has evolved to fit the watery medium in which it developed. Life fits water, rather than the reverse.
Can we imagine life evolving to fit some other liquid, then, one perhaps not too different from water? The obvious candidate is ammonia.
Ammonia is very like water in almost all ways. Whereas the water molecule is made up of an oxygen atom and two hydrogen atoms (H2O) for an atomic weight of 18, the ammonia molecule is made up of a nitrogen atom and three hydrogen atoms (NH3) for an atomic weight of 17. Liquid ammonia has almost as high a heat of evaporation, almost as high a versatility as a solvent, almost as high a tendency to liberate a hydrogen ion.
In fact, chemists have studied reactions proceeding in liquid ammonia and have found them to be quite
analogous to those proceeding in water, so that an "Ammonia chemistry" has been worked out in considerable detail.
Ammonia as a background to life is therefore quite conceivable — but not on earth. The temperatures on earth are such that ammonia exists as a gas. Its boiling point at atmospheric pressure is -33.4° C. (-28° F.) and its freezing point is -77.7° C. (-108° F.).
But other planets?
In 1931, the spectroscope revealed that the atmosphere of Jupiter, and, to a lesser extent, of Saturn, was loaded with ammonia. The notion arose at once of Jupiter being covered by huge ammonia oceans.
To be sure, Jupiter may have a temperature not higher than -100° C. (-148° F.), so that you might suppose the mass of ammonia upon it to exist as a solid, with atmospheric vapor in equilibrium. Too bad. If Jupiter were closer to the sun ...
But wait! The boiling point I have given for ammonia is at atmospheric pressure — earth's atmosphere. At higher pressures, the boiling point would rise, and if Jupiter's atmosphere is dense enough and deep enough, ammonia oceans might be possible after all.
An objection that might, however, be raised against the whole concept of an ammonia background for life, rests on the fact that living organisms are made up of unstable compounds that react quickly, subtly and variously. The proteins that are so characteristic of life-as-we-know-it must consequently be on the edge of instability. A slight rise in temperature and they break down.
A drop in temperature, on the other hand, might make protein molecules too stable. At temperatures near the freezing point of water, many forms of non-warm-blooded life become sluggish indeed. In an ammonia environment with temperatures that are a hundred or so Centigrade degrees lower than the freezing point of water, would not chemical reactions become too slow to support life?
The answer is twofold. In the first place, why is "slow" to be considered "too slow?" Why might there not be forms of life that live at slow motion compared to ourselves? Plants do.
A second and less trivial answer is that the protein structure of developing life adapted itself to the temperature by which it was surrounded. Had it adapted itself over the space of a billion years to liquid ammonia temperatures, protein structures might have been evolved that would be far too unstable to exist for more than a few minutes at liquid water temperatures, but are just stable enough to exist conveniently at liquid ammonia temperatures. These new forms would be just stable enough and unstable enough at low temperatures to support fast-moving forms of life.
Nor need we be concerned over the fact that we can't imagine what those structures might be. Suppose we were creatures who lived constantly at a temperature of a dull red heat (naturally with a chemistry fundamentally different from that we now have). Could we under those circumstances know anything about earth-type proteins? Could we refrigerate vessels to a mere 25° C., form proteins and study them? Would we ever dream of doing so, unless we first discovered life forms utilizing them?
Anything else besides ammonia now?
Well, the truly common elements of the universe are hydrogen, helium, carbon, nitrogen, oxygen and neon. We eliminate helium and neon because they are completely inert and take part in no reactions. In the presence of a vast preponderance of hydrogen throughout the universe, carbon, nitrogen and oxygen would exist as hydrogenated compounds. In the case of oxygen, that would be water (H2O), and in the case of nitrogen, that would be ammonia (NH3). Both of these have been considered. That leaves carbon, which, when hydrogenated, forms methane (CH4).There is methane in the atmosphere of Jupiter and Saturn, along with ammonia; and, in the still more distant planets of Uranus and Neptune, methane is predominant, as ammonia is frozen out. This is because methane is liquid over a temperature range still lower than that of ammonia. It boils at -161.6° C. (-259° F.) and freezes at -182.6° C. (-297° F.) at atmospheric pressure.
Could we then consider methane as a possible background to life with the feature players being still more unstable forms of protein? Unfortunately, it's not that simple.
Ammonia and water are both polar compounds; that is, the electric charges in their molecules are unsymmetrically distributed. The electric charges in the methane molecule are symmetrically distributed, on the other hand, so it is a non-polar compound.
Now, it so happens that a polar liquid will tend to dissolve polar substances but not nonpolar substances, while a nonpolar liquid will tend to dissolve nonpolar substances but not polar ones.
Thus water, which is polar, will dissolve salt and sugar, which are also polar, but will not dissolve fats or oils (lumped together as "lipids" by chemists), which are nonpolar. Hence the proverbial expression, "Oil and water do not mix."
On the other hand, methane, a nonpolar compound, will dissolve lipids but will not dissolve salt or sugar. Proteins and nucleic acids are polar compounds and will not dissolve in methane. In fact, it is difficult to conceive of any structure that would jibe with our notions of what a protein or nucleic acid ought to be that would dissolve in methane.
If we are to consider methane, then, as a background for life, we must change the feature players.
To do so, let's take a look at protein and nucleic acid and ask ourselves what it is about them that makes them essential for life.
Well, for one thing, they are giant molecules, capable of almost infinite variety in structure and therefore potentially possessed of the versatility required as the basis of an almost infinitely varying life.
Is there no other form of molecule that can be as large and complex as proteins and nucleic acids and that can be nonpolar, hence soluble in methane, as well? The most common nonpolar compounds associated with life are the lipids, so we might ask if it is possible for there to exist lipids of giant molecular size.
Such giant lipid molecules are not only possible; they actually exist. Brain tissue, in particular, contains giant lipid molecules of complex structure (and of unknown function). There are large "lipoproteins" and "proteolipids" here and there which are made up of both lipid portions and protein portions combined in a single large molecule. Man is but scratching the surface of lipid chemistry; the potentialities of the nonpolar molecule are greater than we have, until recent decades, realized.
Remember, too, that the biochemical evolution of earth's life has centered about the polar medium of water. Had life developed in a nonpolar medium, such as that of methane, the same evolutionary forces might have endlessly proliferated lipid molecules into complex and delicately unstable forms that might then perform the functions we ordinarily associate with proteins and nucleic acids.
Working still further down on the temperature scale, we encounter the only common substances with a liquid range at temperatures below that of liquid methane. These are hydrogen, helium, and neon. Again, eliminating helium and neon, we are left with hydrogen, the most common substance of all. (Some astronomers think that Jupiter may be four-fifths hydrogen, with the rest mostly helium — in which case good-by ammonia oceans after all.)
Hydrogen is liquid between temperatures of -253° C. (-423° F.) and -259° C. (-434° F.), and no amount of pressure will raise its boiling point higher than -240° C. (-400° F.). This range is only twenty to thirty Centigrade degrees over absolute zero, so that hydrogen forms a conceivable background for the coldest level of life. Hydrogen is nonpolar, and again it would be some sort of lipid that would represent the featured player.
So far the entire discussion has turned on planets colder than the earth. What about planets warmer?
To begin with, we must recognize that there is a sharp chemical division among planets. Three types exist in the solar system and presumably in the universe as a whole.
On cold planets, molecular movements are slow, and even hydrogen and helium (the lightest and therefore the nimblest of all substances) are slow-moving enough to be retained by a planet in the process of formation. Since hydrogen and helium together make up almost all of matter; this means that a large planet would be formed. Jupiter, Saturn, Uranus and Neptune are the examples familiar to us.
On warmer planets, hydrogen and helium move quickly enough to escape. The more complex atoms, mere impurities in the overriding ocean of hydrogen and helium, are sufficient to form only small planets. The chief hydrogenated compound left behind is water, which is the highest-boiling compound of the methane-ammonia-water trio and which, besides, is most apt to form tight complexes with the silicates making up the solid crust of the planet.
Worlds like Mars, earth, and Venus result. Here, ammonia and methane forms of life are impossible. Firstly, the temperatures are high enough to keep those compounds gaseous. Secondly, even if such planets went through a super-ice-age, long aeons after formation, in which temperatures dropped low enough to liquefy ammonia or methane, that would not help. There would be no ammonia or methane in quantities sufficient to support a world-girdling life form.
Imagine, next a world still warmer than our medium trio: a world hot enough to lose even water. The familiar example is Mercury. It is a solid body of rock with little, if anything, in the way of hydrogen or hydrogen-containing compounds.
Does this eliminate any conceivable form of life that we can pin down to existing chemical mechanisms?
Not necessarily.
There are nonhydrogenous liquids, with ranges of temperature higher than that of water. The most common of these, on a cosmic scale, has a liquid range from 113° C. (235° F.) to 445° C. (833° F.); this would fit nicely into the temperature of Mercury's sunside.
But what kind of featured players could be expected against such a background?
So far all the complex molecular structures we have considered have been ordinary organic molecules; giant molecules, that is, made up chiefly of carbon and hydrogen, with oxygen and nitrogen as major "impurities" and sulfur and phosphorus as minor ones. The carbon and hydrogen alone would make up a nonpolar molecule; the oxygen and nitrogen add the polar qualities.
In a watery background (oxygen-hydrogen) one would expect the oxygen atoms of tissue components to outnumber the nitrogen atoms, and on earth this is actually so. Against an ammonia background, I imagine nitrogen atoms would heavily outnumber oxygen atoms. The two subspecies of proteins and nucleic acids that result might be differentiated by an O or an N in parentheses, indicating which species of atom was the more numerous.
The lipids, featured against the methane and hydrogen backgrounds, are poor in both oxygen and nitrogen and are almost entirely carbon and hydrogen, which is why they are nonpolar.
But in a hot world like Mercury, none of these types of compounds could exist. No organic compound of the types most familiar to us, except for the very simplest, could long survive liquid sulfur temperatures. In fact, earthly proteins could not survive a temperature of 60° C. for more than a few minutes.
How then to stabilize organic compounds? The first thought might be to substitute some other element for hydrogen, since hydrogen would, in any case, be in extremely short supply on hot worlds.
So let's consider hydrogen. The hydrogen atom is the smallest of all atoms and it can be squeezed into a molecular structure in places where other atoms will not fit. Any carbon chain, however intricate, can be plastered round and about with small hydrogen atoms to form "hydrocarbons." Any other atom, but one, would be too large.
And which is the "but one?" Well, an atom with chemical properties resembling those of hydrogen (at least as far as the capacity for taking part in particular molecular combinations is concerned) and one which is almost as small as the hydrogen atom, is that of fluorine. Unfortunately, fluorine is so active that chemists have always found it hard to deal with and have naturally turned to the investigation of tamer atomic species.
This changed during World War II. It was then necessary to work with uranium hexafluoride, for that was the only method of getting uranium into a compound that could be made gaseous without trouble. Uranium research had to continue (you know why), so fluorine had to be worked with, willy-nilly.
As a result, a whole group of "fluorocarbons," complex molecules made up of carbon and fluorine rather than carbon and hydrogen, were developed, and the basis laid for a kind of fluoro-organic chemistry.
To be sure, fluorocarbons are far more inert than the corresponding hydrocarbons (in fact, their peculiar value to industry lies in their inertness) and they do not seem to be in the least adaptable to the flexibility and versatility required by life forms.
However, the fluorocarbons so far developed are analogous to polyethylene or polystyrene among the hydro-organics. If we were to judge the potentialities of hydro-organics only from polyethylene, I doubt that we would easily conceive of proteins.
No one has yet, as far as I know, dealt with the problem of fluoroproteins or has even thought of dealing with it — but why not consider it? We can be quite certain that they would not be as active as ordinary proteins at ordinary temperatures. But on a Mercury-type planet, they would be at higher temperatures, and where hydro-organics would be destroyed altogether, fluoro-organcs might well become just active enough to support life, particularly the fluoro-organics that life forms are likely to develop.
Such fluoro-organic-in-sulfur life depends, of course, on the assumption that on hot planets, fuorine, carbon and sulfur would be present in enough quantities to make reasonably probable the development of life forms by random reaction over the life of a solar system. Each of these elements is moderately common in the universe, so the assumption is not an altogether bad one. But, just to be on the safe side, let's consider possible alternatives.
Suppose we abandon carbon as the major component of the giant molecules of life. Are there any other elements which have the almost unique property of carbon — that of being able to form long atomic chains and rings — so that giant molecules reflecting life's versatility can exist?
The atoms that come nearest to carbon in this respect are boron and silicon, boron lying just to the left of carbon on the periodic table (as usually presented) and silicon just beneath it. Of the two, however, boron is a rather rare element. Its participation in random reactions to produce life would be at so slow a rate, because of its low concentration in the planetary crust, that a boron-based life formed within a mere five billion years is of vanishingly small probability.
That leaves us with silicon, and there, at least, we are on firm ground. Mercury, or any hot planet, may be short on carbon, hydrogen and fluorine, but it must be loaded with silicon and oxygen, for these are the major components of rocks. A hot planet which begins by lacking silicon and oxygen as well, just couldn't exist because there would be nothing left in enough quantity to make up more than a scattering of nickel-iron meteorites.
Silicon can form compounds analogous to the carbon chains. Hydrogen atoms tied to a silicon chain, rather than to a carbon chain, form the "silanes." Unfortunately, the silanes are less stable than the corresponding hydrocarbons and are even less likely to exist at high temperatures in the complex arrangements required of molecules making up living tissue.
Yet it remains a fact that silicon does indeed form complex chains in rocks and that those chains can easily withstand temperatures up to white heat. Here, however, we are not dealing with chains composed of silicon atoms only (Si-Si-Si-Si-Si) but of chains of silicon atoms alternating with oxygen atoms (Si-O-Si-O-Si).
It so happens that each silicon atom can latch on to four oxygen atoms, so you must imagine oxygen atoms attached to each silicon atom above and below, with these oxygen atoms being attached to other silicon atoms also, and so on. The result is a three-dimensional network, and an extremely stable one.
But once you begin with a silicon-oxygen chain, what if the silicon atom's capacity for hooking on to two additional atoms is filled not by more oxygen atoms but by carbon atoms, with, of course, hydrogen atoms attached? Such hybrid molecules, both silicon- and carbon-based, are the "silicones." These, too, have been developed chiefly during World War II and since, and are remarkable for their great stability and inertness.
Again, given greater complexity and high temperature, silicones might exhibit the activity and versatility necessary for life. Another possibility: Perhaps silicones may exist in which the carbon groups have fluorine atoms attached, rather than hydrogen atoms. Fluorosilicones would be the logical name for these, though, as far as I know — and I stand very ready to be corrected — none such have yet been studied.
Might there possibly be silicone or fluorosilicone life forms in which simple forms of this class of compound (which can remain liquid up to high temperatures) might be the background of life and complex forms the principal character?
There, then, is my list of life chemistries, spanning the temperature range from near red heat down to near absolute zero:
1. fluorosilicone in fluorosilicone
2. fluorocarbon in sulfur
3.*nucleic acid/protein (O) in water
4. nucleic acid/protein (N) in ammonia
5. lipid in methane
6. lipid in hydrogen
Of this half dozen, the third only is life-as-we-know-it. Lest you miss it, I've marked it with an asterisk.
This, of course, does not exhaust the imagination, for science-fiction writers have postulated metal beings living on nuclear energy, vaporous beings living in gases, energy beings living in stars, mental beings living in space, indescribable beings living in hyperspace, and so on.
It does, however, seem to include the most likely forms that life can take as a purely chemical phenomenon based on the common atoms of the universe.
Thus, when we go out into space there may be more to meet us than we expect. I would look forward not only to our extra-terrestrial brothers who share life-as-we-know-it. I would hope also for an occasional cousin among the life-not-as-we-know-it possibilities.
In fact, I think we ought to prefer our cousins. Competition may be keen, even overkeen, with our brothers, for we may well grasp at one another's planets; but there need only be friendship with our hot-world and cold-world cousins, for we dovetail neatly. Each stellar system might pleasantly support all the varities, each on its own planet, and each planet useless to and undesired by any other variety.
How easy it would be to observe the Tenth Commandment then!
This article is Copyright© 1962 Mercury Press, Inc. Reprinted by permission of the author.
Isaac Asimov is a scientist, a teacher and the prolific author of 225 books to date. Covering a great variety of topics, they range, to mention just a few, from "Biochemistry and Human Metabolism" a textbook (1957), "Races and People" (1955), "The Human Body" (1963), "The Genetic Code" (1963), "Intelligent Man's Guide to Science" (1960), "Short History of Chemistry" (1965), "Quick and Easy Math" (1964), "Great Ideas of Science" (1969) and "Our World in Space" (1974) to "Asimov's Guide to the Bible" in 2 volumes (1968-1969), "Asimov's Guide to Shakesspeare" in 2 volumes (1970), Asimov's Annotated Paradise Lost" (1974) and "Isaac Asimov's Treasury of Humor" (1971). His accompanying article is from his book "View from a Height" (1963).
Born in Russia in 1920, Isaac Asimov came to the U.S. at the age of 13. He holds B.S., M.A. and Ph.D. degrees from Columbia University.
Dr. Asimov received the James T. Grady award of the American Chemical Society in 1965 and the American Association for the Advancement of Science — Westinghouse Science Writing Award in 1967. Dr. Asimov has been with the Boston University School of Medicine since 1949 and a member of its faculty since 1955.
|
In Which Klingons Became Chimeras
By: Frank Drake
SETI has recently been afflicted by a serious disease, a disease curable through the steady application of good old horse sense. I'll call it Star Trekitis, because the first germs were spread by Capt. Kirk and company along with a very laudable awakening in awareness of the fascinating richness of space phenomena. The symptoms are frequent calls from media reporters posing statements such as "I hear that the failure of your searches for signals means that we are alone in the galaxy," or, worse, "I understand that because 'They' are not here, it has been proven that we are alone in the galaxy." The debilitating effect of the disease is concern among those who provide funds for SETI as to whether any further support is justified.
Now the first symptom is easily cured. It is easy to show that the searching which has been done, and it now amounts to an impressive tens of millions of tests of frequencies and directions in space, is still a drop in the bucket. In the immensity of the cosmic haystack, it will take many many more trials than those to create a reasonable chance of success. The program now being developed in NASA addresses this very problem, and we can hope that the effect of Star Trekitis does not delay it.
The second symptom is the provocative one. Its roots he in a seductive logic which is impressive, but nonetheless is a classic case where logic and common sense don't reach the same conclusion. The logic goes like this: Eventually every technical civilization develops the ability to send spacecraft to other stars. They will then send colonizers to suitable planets of nearby stars. In time each colony will develop a space industry able to launch its own colonies. In some finite time this colonizing civilization will reach and colonize every suitable planet in the galaxy.
Now the punch line is that even the most conservative estimate of the finite time for total colonization of the galaxy is only about 100 million years, an instant in the age of the galaxy. It all sounds like a typical Star Trek scenario — yes, it says, there should be a Klingon Empire out there. Most importantly, the first civilization to colonize inherits the galaxy, which is pretty heady stuff. No one else has a chance. The logical conclusion: Since no Klingons of any sort have come to earth, we must be the first — and maybe only — intelligent creatures in the galaxy. Therefore, searching for other civilizations is a waste of time and money.
The flaw in this is the tacit assumption that anything which is possible will happen. This theorem does seem to apply to natural phenomena in space, but now we are talking about intelligent creatures. They do not do everything possible, but only that which has the biggest payoff. Look at us: we could build a building a mile high (Frank Lloyd Wright designed one), but we don't. We built a few Concordes before realizing the payoff wasn't there, at which point the project was abandoned.
Now if there is anything which doesn't provide an attractive payoff, no matter how rich you are, it is interstellar colonization. The simplest of physical laws and the vast interstellar distances force any colonization scheme to be preposterously expensive. Here again Star Trek has led us astray with its one hour journeys from star to star. For example, if we launched one hundred colonists in a spacecraft providing a mass of ten tons per colonist (only a little more than the typical mass per passenger in a large jet plane), at a speed which would carry the spacecraft only ten light years in 100 years time, what is the absolute minimum amount of energy required? Answer: the same amount as 400,000 people consume in all ways in their lifetimes! Putting in some reasonable efficiencies for fuel production, etc. the minimum amount of energy grows to equal the total energy consumption of the United States for 100 years! Would you shut down America for a hundred years to launch a colony on a very iffy mission which would do you no good? Would any government do that?
How about the even more costly round trip missions? Barney Oliver recently worked out the minimum energy required to send a 1000 ton payload to Alpha Centauri and back in 50 years. It turns out to equal 4000 years of the total U.S. electrical energy production. Even assuming 100 percent efficiency, the cost at current rates would be 400 trillion dollars, which would affect income tax rates in a rather depressing way.
The point is that even if you had funds like this, you would spend them in more beneficial ways. For far less cost per person, you could provide a good life to countless people by floating cities on the oceans or turning the arctic into a Garden of Eden. If you are intent on providing a good life to ever more people, you are far better off to pursue the building of space colonies as proposed by Gerard O'Neill. Yes, there may be great technologies; there may be countless planets which have been reformed into vast, comfortable habitats; there may even be fleets of space colonies orbiting other stars. But no Klingon Empires. Despite widespread intelligence and wealth in space, no Mayflower will descend from the sky.
Intelligent civilizations will limit their contacts to intellectual ones, touching one another, we reason, through radio and other electromagnetic waves. This is the ultimately logical way for logical beings. We should proceed with our radio searches.
Dr. Frank D. Drake is Director of the National Astronomy and Ionosphere Center of Cornell University which includes the Arecibo Observatory. Dr. Drake is a member of the Editorial Board
of COSMIC SEARCH.
|
Universal Chemical Evolution The Chemistry of Space
By: Robert H. Rubin
Introduction
When wondering what extra terrestrial intelligent life — or just life — will be like, we must consider its chemistry. Here on earth life is based on organic chemistry — that is carbon chemistry. In our assessments of what may be elsewhere in the universe it is necessary to consider other possible cosmic bio-chemistries than the one case we know here at home. Many aspects of the other-life-in-the-universe issue turn out to produce criteria that are surprisingly similar to those on earth. By considering the stable lifetimes of stars and the potential habitable zones around them, it turns out that the best candidate stars for life bearing planets are stars very similar to the sun. (See COSMIC SEARCH, Vol. 1, No. 3, Summer 1979, page 36).
Another case in point arises when we consider the best way to communicate with Extra-Terrestrial Intelligence (ETI). Many workers feel the answer is by radio waves in the frequency range between 1.42 and 1.72 gigahertz (see COSMIC SEARCH Vol. 1, No. 1, page 35). This is the so-called "water hole" since it is flanked by lines of hydrogen (H) and the hydroxyl radical (OH), the combination of H and OH being H2O or water. On the earth water is inextricable from life. Without arguing the merits of the conclusion that the water hole is the best region, here too we see, as in the case of the sun-like star of the first example, an anthropocentric view is sustained, that life elsewhere should be like it is here. While speculation in this field is certain, a careful scientific examination is necessary to limit the multitudinous possibilities and enhance our chances of success in searching for ETI. It is in this spirit that we shall address the issue of cosmic chemistry.
The Elements from Hydrogen through Oxygen
An introduction to the elements
|
Atomic number | Element | Symbol | Electrons | Atomic weight* | Nucleons** |
1 | Hydrogen | H | 1 | 1.008 | 1p |
2 | Helium | He | 2 | 4.003 | 2p + 2n |
3 | Lithium | Li | 2 + 1 | 6.940 | 3p + 4n |
4 | Berylllium | Be | 2 + 2 | 9.013 | 4p + 5n |
5 | Boron | B | 2 + 2 + 1 | 10.82 | 5p + 5n |
6 | Carbon | C | 2 + 2 + 2 | 12.011 | 6p + 6n |
7 | Nitrogen | N | 2 + 2 + 3 | 14.008 | 7p + 7n |
8 | Oxygen | O | 2 + 2 + 4 | 16.000 | 8p + 8n |
*Based on atomic weight of oxygen equal to 16.
**Nucleons: constituents of the nucleus of the atom (protons, p, or neutrons, n).
In the last decade or so much new information has been learned that has a direct bearing on universal chemistry. This has come from various astronomical observations. The chemistry laboratory now includes meteoroids, comets, and interstellar gas and dust clouds.
Meteorites
For over a hundred years it has been suspected that complex organic matter is present in meteorites. When the Orgueil meteorite that fell in France in 1864 was claimed to contain amino acids (the building blocks of proteins), the scientific community was not sympathetic, claiming it was contaminated by earthly organics. Since the meteorite had been displayed many years in the Museum of Natural History in Paris probably undergoing a regular dusting that necessitated touching (and since finger prints transfer amino acids) the stone surely was not a good case for extra-terrestrial amino acids. In fact, the percent of the various amino acids in finger prints and the meteorite were similar!
The modern era of meteorite analysis was ushered in with the work of Cyril Ponnamperuma and his associates. This was aided greatly by "clean room" techniques developed for the analysis of returned lunar rocks. The type of meteorite of particular interest is the carbonaceous chondrite. The former part of the name is due to the fact they are the richest of the meteorites in carbon content (as high as 5 percent by weight). The latter part is because they have glass like spheres called chondrites about 1 to 10 millimeters in diameter. They are also copious sources of water, some containing more than 10 percent by weight. Ponnamperuma and his collaborators, then working at NASA Ames Research Center in California, first analyzed the Murchison meteorite, a carbonaceous chondrite, which fell September 28, 1969 near Murchison, Victoria, Australia. Stones were picked up soon after the fall, those least cracked were culled out, and an interior piece pulverized and analyzed within a few months after its fall. The chemical identification was performed using a gas chromatograph combined with a highly sensitive mass spectrometer. In their initial publication they report finding 5 amino acids that are also found in living things and 2 not found in biological systems. Additional work and a later report increased these to 6 and 12 respectively. This is supportive of the hypothesis that the amino acids must have been synthesized non-biologically. The researchers also were able to determine for several the percentages of left-handed (L) and right-handed (D) amino acids. The terms right and left handed refer to the fact that the amino acids differ only in that they are mirror images of each other like a left and right hand. All the amino acids with the exception of the simplest, glycine, show this effect. Glycine's mirror image can be rotated to superimpose exactly with the original. In living systems on earth, all amino acids other than glycine are L type (except the case of a class of single cell organisms that are made of D type in their cell walls). In the Murchison meteorite they found about equal amounts of both L and D type. Together with the above fact that most of the amino acids in the meteorite are not of the type associated with living things strongly suggests all of the amino acids present were produced before they arrived on earth. The final clincher was the ratio of carbon 13 to carbon 1 [sic; should the latter be "12" instead of "1"?] was significantly higher in the organic matter in the Murchison than in terrestrial organic matter. All the evidence points to the indigenous nature of the hydrocarbons and amino acids in the meteorite.
In the early morning hours of February 8, 1969 the Allende meteorite put on a spectacular display appearing brighter than a full moon as it plummeted to the ground near the northern Mexico village for which it is named. Within days much material was collected from this fall. An analysis similar to that performed on the Murchison produced similar results. The experimenters also did not have the word "contamination" thrown up as a criticism as in the early days of meteorite analyses, since
the stones were found so soon.
Most recently, Ponnamperuma (now at the University of Maryland) and his colleagues have examined two carbonaceous chondrites from Antarctica — one called Yamato 74662 and the other called Allan Hills. Again they found lots of amino acids (both proteinaceous and non-proteinaceous) containing roughly equal amounts of D and L type. These meteorites appear to be uncontaminated by organic matter from the Earth. Indeed, they show less contamination than did the Murchison even though they fell about 200,000 years ago! This is deduced from the fact that there are equal amounts of amino acids in samples taken from the interior and the exterior. Specifically, in Yamato 74662, 15 amino acids were detected including 9 biological and 6 non-biological while the Allan Hills meteorite had 6 biological and 5 non-biological amino acids. As with the other analyses, the most recent research on the Antarctic meteorites suggests the organic matter including the amino acids are of extra-terrestrial origin.
Meteorites are rocks probably from the asteroid belt or comet remnants that fall to the earth. They are believed to be as old as the solar system having formed about 4.6 billion years ago from the solar nebula. The substances found in meteorites give clues to the kind of chemical reactions that are possible in a system other than Earth's. In addition they may provide vital clues to what processes occurred here on Earth prior to the beginning of life. As far as cosmic chemistry is concerned, the complex molecules that evolved in the meteorites are organic molecules that evolved also on earth. The complex molecules contain for the most part the elements carbon, hydrogen, nitrogen, and oxygen — precisely those atoms most abundant in living things. These are also, in terms of number of atoms, the most abundant elements in the universe — with two exceptions. Helium is the second most abundant element and neon is close to carbon, nitrogen and oxygen in rank. However both helium and neon are inert as a result of full outer electron shells and they don't form molecules (except in rare cases).
Comets
Comets are another source of clues to the understanding of cosmic chemical evolution. Comets, even more than meteorites, provide a sample of the solar nebula which is well preserved. We estimate that the mass of a comet is not very large, perhaps typically the mass of an earth mountain. We have yet to visit a comet although NASA would like to do so when Halley's comet returns in 1985/1986. Still, the chemical analysis can be done remotely from earth by spectroscopy. Optical astronomers have known for many years that comets contain relatively simple diatomic and triatomic molecules (see Table 1). Some are organic since they contain carbon. More recently radio astronomers have been able to extend the list of these molecules by identifying additional substances from their radio lines.
TABLE 1
MOLECULES IDENTIFIED IN COMETS
|
Name of molecule | Chemical Symbol |
Hydroxyl radical | OH |
Methylidyne | CH |
Cyanogen radical | CN |
Ionized hydrogen | H2+ |
Ionized nitrogen | N2+ |
Carbon | C2 |
Carbon monosulphide | CS |
Amide | NH2 |
Ammonia | NH3 |
Carbon monoxide | CO |
Carbon dioxide | CO2 |
Water | H2O |
Hydrogen cyanide | HCN |
Methyl cyanide | CH3CN |
Comet Kohoutek in 1973/1974, which turned out to be such a disappointment to the general public after an unprecedented TV and press build up, actually proved to be very fruitful for scientists. Radio molecular spectroscopists believe they have indentified hydrogen cyanide (HCN) and the most complex compound yet found in comets, methyl cyanide (CH3CN), being emitted by this comet. Calculations of the orbit of comet Kohoutek show that it spends most of its time well beyond the orbit of Pluto in the frozen depths of space. As it approaches the sun under the influence of gravity, the frozen substances can vaporize. It is this gaseous component (often called the coma that surrounds the solid head) that radio astronomers look for with their telescopes. Most probably organic compounds like methyl cyanide are present in the comet throughout its journey around the sun. Comets most likely formed from the nebulosity creating the sun, the planets, and the asteroids and are therefore as old as the solar system itself. It is possible that the molecules in Table 1 are decomposition products of larger parent molecules which break down when exposed to the intense solar radiation.
Astronomers are anxiously awaiting the appearance of a very bright comet. A bright optical comet also will likely be the best candidate to identify molecules by their radio lines. As will be discussed in the next section, there are many complex molecules radio astronomers have found in the interstellar medium. These undoubtedly would be high on the list of priorities to look for in bright comets. Whether Halley's comet will be bright enough for such observations in 1985/86 is not certain. There is also a proposal of a second mission to rendezvous and follow comet Temple II in 1988/89 while close to the Sun. This would provide a dynamic picture of the changing chemistry as the solar radiation and wind vary. There is even the exciting possibility of landing on the head and making direct chemical analyses! Or eventually the "comet of the century" will appear that is bright enough for detection and analysis of many more substances. The indication is that, like meteorites, the complex molecules will be organic and composed of predominantly C, H, N, and O.
The Interstellar Medium
On a much grander scale than comets or meteorites we are now able to say quite a lot about the chemistry of interstellar space, that is the region of the galaxy that is between the stars. It is mainly gas and dust. Just a little over a decade ago it was thought that nothing more complicated than a diatomic molecule would be found in these regions. The reasoning then was that collisions between atoms in the extreme vacuum of the nebulae (clouds of gas and dust in space) would be so rare that gas phase reactions would build molecules of no more than two atoms. The revolution began with the radio astronomy detections of ammonia and water by their microwave spectral lines. In just over a decade, the list of molecules found in the interstellar medium has grown to over 50, and this does not include isotopic variations of the molecules. In Table 2 we list those species detected up until August, 1979, the time of the International Astronomical Union symposium on interstellar molecules. Ozone, O3, was discovered after that meeting. It's addition makes Table 2 complete as of January, 1980. Most of these are carbon containing organic molecules. Many of these molecules will be recognized as having appeared in comets — CH, CN, OH, CO, C2, CS, H2O, HCN, NH3, and CH3CN. The heaviest molecule and also the one with the most atoms yet found in interstellar space is cyano-octatetra-yne HC9N. It contains 11 atoms and has a molecular weight of 123. It is interesting that although no amino acid has yet been found in space, the simplest one, glycine, has less atoms (10) and is lighter (molecular weight of 75) than HC9N. By far the largest number of compounds in Table 2 contain, H, C, N, and O; ten contain sulfur, and two have silicon. Even though the list of molecules is not a random sample, the picture is quite clear that the most abundant elements in these molecules of interstellar space are the same as in living things on earth. Sulfur also is essential for all terrestrial life forms. It is the only element besides H, C, N, and O that is incorporated into amino acids needed for life — cysteine and methionine.
TABLE 2
MOLECULES DETECTED IN INTERSTELLAR CLOUDS
The order is approximately by time of discovery.
|
Name of molecule | Chemical Symbol |
Methylidyne | CH |
Cyanogen radical | CN |
Methylidyne ion | CH+ |
Hydroxyl radical | OH |
Ammonia | NH3 |
Water | H2O |
Formaldehyde | H2CO |
Carbon monoxide | CO |
Hydrogen cyanide | HCN |
Cyanoacetylene | HC3N |
Hydrogen | H2 |
Methyl alcohol | CH3OH |
Formic acid | HCOOH |
Ionized formyl radical | HCO+ |
Formamide | NH2COH |
Carbon monosulfide | CS |
Silicon monoxide | SiO |
Carbonyl sulfide | OCS |
Methyl cyanide | CH3CN |
Isocyanic acid | HNCO |
Methylacetylene | CH3C2H |
Acetaldehyde | CH3CHO |
Thioformaldehyde | H2CS |
Hydrogen isocyanide | HNC |
Hydrogen sulfide | H2S |
Methanimine | H2CNH |
Sulfur monoxide | SO |
Protonated nitrogen ion | N2H+ |
Ethynyl radical | C2H |
Methylamine | CH3NH2 |
Dimethyl ether | (CH3)2O |
Ethyl alcohol | CH3CH2OH |
Sulfur dioxide | SO2 |
Silicon sulfide | SiS |
Acrylonitrile | H2CCHCN |
Methyl formate | HCOOCH3 |
Nitrogen sulfide radical | NS |
Cyanamide | NH2CN |
Cyanodiacetylene | HC5N |
Formyl radical | HCO |
Cyanoethynyl radical | C3N |
Acetylene | C2H2 |
Cyanotriacetylene | HC7N |
Ketene | H2C2O |
Nitroxyl | HNO |
Ethyl cyanide | CH3CH2CN |
Carbon | C2 |
Cyano-octatetra-yne | HC9N |
Methane | CH4 |
Nitric oxide | NO |
Butadiynyl | C4H |
Methyl mercaptan | CH3SH |
Isothiocyanic acid | HNCS |
Ozone | O3 |
What allows these complex molecules to form in the seemingly harsh environment of space? What the astrochemists of the pre-molecule explosion had overlooked was the important role of dust grains in the formation of molecular hydrogen. Also in regions of much higher than average density, the densest clouds, collisions in the gas phase are not so infrequent that more complicated molecules could not be formed. This is aided greatly by reactions between ions and molecules. The energetic
radiation present can ionize some of the atoms and molecules in the cloud. It is much easier then for a positively charged atom or molecule to interact with a neutral one than if both were uncharged. The reason is that as two neutral atoms or molecules approach each other, they first feel the electrostatic repulsion of their electrons. This acts as a sort of shield to ward off the advance of the other. When one of them is an ion however, this shielding is much reduced and could even be an electrostatic attraction of a net positive to a negative charge.
Carbon Chauvinism
Why does carbon play such an important role in cosmic chemistry? Could another element serve the same function and be the basis of life elsewhere? An important reason for the ability of carbon to form molecules is that is has four chemical bonds, the most possible for any atom. We say carbon has a valence of four. The only other element with the same capability that is at all cosmically abundant is silicon. Silicon is about 25 times less abundant than carbon (by number of atoms) in the universe, but it is more than 100 times as abundant on earth. Strictly from this standpoint certainly the earth would be a good candidate for a silicon rather than a carbon based life.
In terms of chemical bonding there are several arguments that make the case for a silicon-based life extremely weak. The Si-Si bond (42 kilocalories mole)* is only about half that of the C-C bond (80 kilocalories/mole) which means it is easier to break. (*A mole equals the gram molecular weight which equals the weight (in grams) of the total of the atomic weights of the atoms making up the molecule. Thus, a mole of carbon monoxide (CO) is 12.011 + 16.00 = 28.011 grams. — Ed.) Also silicon bonds very strongly with oxygen. In the presence of hydrogen, oxygen, or other silicon atoms, silicon will overwhelmingly choose oxygen. Since oxygen is about 25 times as abundant as silicon cosmically, the chances are most of the silicon will be used up in combining with oxygen. This is supported by the silicon compounds present on earth as well as those observed in interstellar space — SiO and SiS (sulfur is below oxygen in the periodic table so is chemically similar). Also the Si-Si bond is unstable in the presence of liquid water or ammonia. On the other hand for carbon, the C-C bond is of comparable strength to the C-O and C-H bond allowing the formation of carbon ring structures that are characteristic of important pre-biotic molecules and appear in the bases of DNA. Since the Si-O bond is stronger than the Si-Si bond, the formation of Si ring structures is improbable. Furthermore, silicon does not usually make multiple bonds while carbon often does, leaving carbon free to react with other atoms while hanging onto the structure it already has.
Another important comparison is between the oxides CO2 and SiO2. Carbon dioxide is gaseous (and thus free to associate with many other substances) for any reasonable temperature for which we believe life will exist, while silicon dioxide is a solid quartz at these temperatures. CO2 is soluble in water and relatively easy to dissociate while SiO2 is insoluble in water or most anything and is ultra hard to break up. Imagine the difficulty the photosynthesis process, vital to life on earth, would have with SiO2. Finally, we introduce perhaps the most decisive evidence of all. For whatever reasons, carbon does make more compounds by far than all of the other elements combined do in non-carbon compounds! This is the case not only in the earth laboratory but also in meteorites, comets, and interstellar clouds.
What might happen or have happened elsewhere in the Universe regarding the subsequent evolutionary steps — let's say the biochemical evolution — is another story. Right now this is a more speculative area than what we have discussed here. Probably most of the experts would say that the omnipresence of DNA in life on earth would not extend to the Universe. Nature could more than likely create other self replicating molecules that would play a surrogate role for DNA elsewhere. However, most workers in the field would most certainly bet that the overwhelming majority of extraterrestrial life forms are C, H, N, and O-based.
Robert H. Rubin is a member of the physics faculty of the California State University at Fullerton. Dr. Rubin received his Ph.D. degree from Case-Western Reserve University (Cleveland, Ohio).
|
Letters:
By: Editors
Letters are always welcome but owing to the volume it is not possible to acknowledge all of them. Also due to space limitations we reserve the right where necessary to condense or edit the contents. Letters may be addressed to: Editorial Dept., COSMIC SEARCH, P.O. Box 293, Delaware, Ohio 43015.
Most discussions of SETI assume that the primary topic of interstellar conversations will be science, but this may be a somewhat provincial assumption, reflecting only that the primary interest of most people involved in SETI is science. Of course, civilizations that broadcast interstellar signals are going to know a lot of science, but science may not be their primary interest. Our radio telescopes may not receive the science of many worlds, but the religions.
On Earth today people devote far more energy to religion than to science. The airways are full of religious programming, while science programming is relatively scarce. The same may hold true for the interstellar airways. Though many people assume that as a species develops science it leaves religion behind, this is not necessarily so. We may find that interstellar communication consists largely of thousands of worlds trying to make converts of each other.
It is always possible that everyone else in the universe will turn out to be Christians or Buddhists or Hindus, and that this will be considered proof of the validity of whichever religion is the religion of the cosmos, but it seems more likely to expect a bewildering diversity of religions — and this may cause major problems.
Over the past few centuries westerners have been exposed to eastern religions, and vice versa, and the result has brought self-doubts and conversions on all sides. Can you imagine what would happen if humans suddenly had not five or six major religions to choose from, but thousands, each backed up by the prestige of a great civilization, many of them far more advanced — both scientifically and psychologically — than ourselves? The result might be social chaos. Humans might decide that among so many religions our own couldn't possibly be correct, and they would adopt extraterrestrial religions, each one of which might call for different ethical values and social behaviors. These contradictory behaviors alone could cause serious conflicts. Let us not forget that humans have fought many wars because two groups merely believed in different religions.
This possibility may mean that few civilizations in the universe are searching for interstellar signals. Perhaps after a bad experience with social chaos, civilizations will cut themselves off from the universe. Or, if they go on listening, the radio telescopes will be controlled by a priesthood that censors everything dangerous, and also broadcasts their sacred texts to convert other civilizations to their religion.
Of course, all of this is highly speculative. It may turn out that only humans have religions. And even if religion is widespread, the radio telescopes of other worlds may be, as on Earth, in the hands of creatures whose primary interest is science. But if interstellar communication turns out to be primarily religious in content, civilizations will still exchange a lot of science; it is just that reports on the geology of a solar system will be inserted between sacred texts.
Don Lago
Columbia, Missouri
•
Congratulations on publishing such a fine journal. I have thoroughly enjoyed the issues and have used some of the material to pique the interest of my young students.
I suggest a plan be formulated outlining how Amateur Radio operators might support SETI, and the plan be submitted to the Amateur Radio press for publication. I believe you might find fertile ground among this world-wide inquisitive and innovative fraternity.
Nelson Lecklinkner
N6AQY
Novato, California
Radio amateurs could well be interested because an extraterrestrial intelligence could provide the ultimate DX contact. — Ed.
•
Thank you for the uniform excellence of your publication. The ABCs of Space combines brevity with accuracy and excitement, a combination as elegant as it is rare. It has inspired me to work my way through a college-level astronomy text.
Your magazine sometimes lists materials, such as government documents, which are hard to find. Where may I obtain them?
Joe W. Morganti
Berkely, California
U.S. Government publications can generally be obtained from the U.S. Government Printing Office, Washington, D.C.
Most large cities in the U.S. have a Bookstore of the Government Printing Office. Check your telephone directory. Prices are very reasonable. — Ed.
•
Although James Elliot of Cornell has contributed to the consideration of lunar or artificial satellite occultations combined with Space Telescope observations for planet detection purposes, he is not the originator of the idea, as suggested by the SEnTInel in the Summer 1980 COSMIC SEARCH. In fact, he might be considered a "Jimmy-come lately" to these considerations.
To my knowledge, the idea was proposed by Dr. Nancy Roman of NASA in Astronomical Journal, 64, No. 1273, 344-345, October 1959 and was further developed by L. Spitzer Jr. in American Scientist, 50, 473-484, Sept. 1962. The author of this letter, in collaboration with A.J. Fennelly and G. Frye has discussed lunar occultation/Space Telescope extra-solar planet detection in Journal Optical Society of America, 64, 531, 1974 and JBIS, 28, 399-404, June 1975. A review of this earlier work by C.E. Kenknight appeared in Icarus, 30, 422-433, 1977.
Now that I've gotten this critique off my chest, I'd like to comment that COSMIC SEARCH is a most splendid magazine. Keep up the good work!
Dr. Gregory L. Matloff
Brooklyn, New York
Thank you for this added information and perspective. Interested readers may find your references very useful. We regret any unintended implications. — RSD
Regardless of who proposed the planet detection idea, Elliot has used the occultation method effectively in other ways, as for example in observing the atmosphere of Mars during its occultation of Epsilon Geminorum, and in his discovery of the rings of Uranus. He is continuing this work and plans to make occultation observations of the rings of Saturn. For his observations Elliot has used NASA's Kuiper Airborne Observatory, a large 4-engine jet aircraft. — JK
•
Referring to the article by Pasachoff and Kutner in "Neutrinos for Interstellar Communication" in the Summer 1979 issue of COSMIC SEARCH please be advised that the possible use of neutrino beams for telecommunication not only over global but also interstellar distances was investigated and suggested in my papers, published 13 years earlier in Polish scientific journals.
I investigated the neutrino beams not only from accelerators but also from the radioactive atomic nuclei polarized in very low temperatures in magnetic fields. In the case of the accelerator neutrinos I have taken into the account the protons of 25 GeV with the neutrino beam angles 3° and 20' and protons of 300 GeV with neutrino beam angles 1°, 1', and 1". It was accepted that the accelerator generates pulsed beam of 1015 protons and a pulse rate is equal 103 per hour. Because of the parity non-conservation in weak interactions one can get also oriented neutrino emission from the polarized nuclei. For both cases the neutrino fluxes were calculated for interstellar distances.
This will enable the start of neutrino astronomy and the verification of the possible neutrino channel used by ETI. Obviously, sending and detection of the signals over global distances (through the Earth) will be much simpler. The main results of my papers /Subotowicz, 1967/ I have presented for the second time during the 25-th International Astronautical Congress in Amsterdam, 1974. There presented paper was published recently in "Astronautica Acta," 1979. My earliest papers on this subject /Subotwicz, 1967, were mentioned in several international abstracts and bibliographies. All this is described in my paper published in "Postepy Astronautyki," Subotowicz, 1979.
References:
Subotowicz M. (1967), Neutrina w lacznosci miedzygwiezdnej (in Polish) - Neutrinos in the interstellar communication, POSTEPY TECHNIKI JADROWEJ (Progresses in nuclear Techniques), 11, 475-479
Subotowicz M. (1979), On the communication with neutrino beams. POSTEPY ASTRONAUTYKI (Progresses in Astronautics), 12, No 3, 153-156
Prof. Dr. M. Subotowicz
Institute of Physics
M. Curie-Sklodowaska University
Lubin, Poland
|
College Courses on "Life in the Universe"
By: Editors
College courses on "Life in the Universe" are becoming increasingly popular. Although dealing mainly
with astronomy, many of the courses include much interdisiplinary material from such diverse areas as biology, medicine, psychology, physiology, semantics and communication.
We would like to include additional institutions. Please send information to Prof. John Kraus, Ohio State University Radio Observatory, 2015 Neil Ave., Columbus, Ohio 43210. Years given, attendance, a syllabus (if available) and other information will be appreciated.
In previous issues we have listed 25 colleges or universities offering or planning to offer courses. We list here 4 more institutions: University of Innsbruck, Austria; University of Missouri (Columbia); North Seattle Community College, Washington; and the Far East Division of the University of Maryland.
Institution: Astronomical Institute, University of Innsbruck, Austria, 6020.
Instructor: Dr. Georg M. Gruber (on leave from Rhodes University, Grahamstown, South Africa).
Title: Possibilities of Life in the Universe.
Content: Basic astronomy, evolution of life, interstellar communication and colonization.
References: Goldsmith and Owen, Butler and Messel, Firsoff, COSMIC SEARCH.
Years given: 1981
•
Institution: University of Missouri (Columbia) 65211.
Instructor: Prof. Charles J. Peterson, Dept. of Physics and Astronomy.
Title: SETI
Content: Life as we know it, planetary systems, the search for extra-terrestrial civilizations.
References: Bracewell, "The Galactic Club;" Goldsmith and Owen, "The Search for Life in the Universe;" COSMIC SEARCH, Icarus, Mercury.
Years given: 1980
Enrollment: 15
•
Institution: North Seattle Community College, Seattle, Washington 98103.
Instructor: John E. Baer, Division of Science and Mathematics.
Title: Life in the Universe.
Content: A survey of our universe, focusing on the probability of life elsewhere, the search for alien civilizations and the future of civilizations, including our own.
References: Goldsmith and Owen, "The Search for Life in the Universe;" O'Neill, "The High Frontier;" COSMIC SEARCH.
Year given: 1980.
Enrollment: 24
•
Institution: University of Maryland, Far East Division, APO San Francisco, California 96328.
Instructor: Dr. Peter M. Kranz
Title: Extraterrestrial Life and Interstellar Travel
Content: Origin of life, stellar and planetary evolution, the search for extraterrestrial life.
References: Hoyle, "Black Cloud;" Sagan, "Cosmic Connection;" Ridpath, "Worlds Beyond;" Wald,
"Origin of Life;" Jastrow and Thompson, "Fundamentals of Astronomy".
Years given: 1980
Enrollment: 30
|
ETI in the Classroom
By: Philip E. Barnhart
For some years science fiction has served as a lure to attract the college student seeking to expand upon a traditional experience in literature or basic communications. Such courses seem popular and students evaluate them as meaningful experiences, but as a teaching scientist I avoid using fiction for two reasons: (1) much time is spent in dispelling gimmickry and (2) my experience with non-fictional science leads me to conclude scientists are far more imaginative and creative than non-scientist writers of fantasy and fiction.
The coming of age of exobiology with the acceptance of a serious search for evidence of ExtraTerrestrial Intelligence (ETI) meant that I had a real subject with which to hook the imagination of curious, motivated students. Although contact with ETI is not a fait accompli, the history of science abounds with protracted searches for predicted phenomena. Stellar parallax, a direct consequence of the Copernicus heliocentric model of the solar system, eluded observers from the latter part of the 16th century until 1838, yet many valuable and meaningful discoveries were made as a result of the search. The neutrino, postulated in 1933 eluded detection until 1956. Isolated quarks may never be found. The grand achievements of science come only with the search.
My awakening to the possibility of appealing to the natural urge on the part of students to explore beyond the bounds of their provincial existence came with my first reading of Shklovski and Sagan's "Intelligent Life in the Universe." In this work I was introduced to the almost trivial exercise devised by Frank Drake to illustrate how radio transmission could allow communication with a civilization having no possibility of linguistic interchange. The only common bond would be experience with the same cosmic environment. The common language must be the science and logic needed to comprehend the cosmos to the extent necessary to build instruments capable of exchanging communication. I, at least, was hooked.
I had to play with the idea for a while. My experience with ETI, coding, anti-coding, searching, interpreting and believing were as fresh and untried as would be that of my students. I started slowly; making slides of the Drake message, lecturing to local groups about "Communicating with ETI," slipping a lecture here and there into courses in introductory astronomy, beginning physics and faculty seminars. I began to see where the interest and fascination came during the presentations.
I even recorded the Drake message as a two-tone beep signal on magnetic tape. A series of 1 second tones separated by 1 second intervals requires 18 minutes and 20 seconds recording time. I then speeded it up by a factor of 64 so the entire message requires just over 20 seconds to play and comes out as an unrecognizable whistle.
A final step of adding a large quantity of random noise gives me the opportunity to introduce the whole process, from signal search through noise suppression to analysis. The detection of a noisy radio signal provides an opportunity to illustrate the nature of measurement at the threshhold of detectability. The simulated computer processing to remove the non-random signal from the noise (accomplished on the master tape by suitably adjusting the gain downward on the random noise and the gain upward on the Drake signal (a process I call "manual signal averaging"), and manipulation of the playback rate puts the Drake signal in a form recognizable as intelligently generated. It is also subject to transcription by moderately adept students.
The student, when presented the puzzle of the Drake message in this way, develops a heightened fascination with possible content. I find students devoting the same time and effort to the solution as they invest in computer games and racquetball. The next step in using the Drake type of message will be an interactive program in which a variety of messages can be introduced at a terminal so a student may employ a variety of strategies to find and process anti-coded messages.
After the Drake picture is rendered, whether by individual students or the instructor, the class is invited to produce interpretations of the message content. There exists in Shklovski and Sagan a fairly complete description of Drake's original ideas with which to compare different attempts to decide what the message communicates. In a typical class it is not unusual to find conceptualization ranging from a cartoon-type portrayal with dialog balloons, through sexual fantasy to speculation about galactic evolution. Class discussion to resolve differences in interpretation produces fascinating insight into the processes and information utilized by college students to solve problems.
I have found most of my students are unfamiliar with the early Drake message. Many have encountered similar demonstrations generated at later times but practically none have bothered to delve into any meaning contained in the messages. Both the Drake message and Sagan's Pioneer plaque provide departure points for various scientific ideas. Each provides a substantial case for a "need to know," especially of the mathematical and astronomical fundamentals.
After using the Drake message for brief periods in several classes I began to explore the possibility of expanding the study of ETI to a full unit course. At Otterbein College we have a program of composition and literature courses spread throughout the student's entire college career. During the first term in college a student may select a topical seminar as a means of fulfilling the introductory composition and literature requirement.
These Freshman Seminars are taught by faculty from all divisions of the college. Subject matter usually centers about a special interest of the instructor. Typical subjects encountered are ecological awareness, stress management, the study of ghosts, and utopias. Among the objectives of the seminars are the development of communication and study skills along with the discovery of research tools available on the college campus. A substantial amount of writing experience is required and growth in this area is expected.
To me, the concept of communication as a raw, theoretical idea can best be explored in the context of communication with an intelligent extra-terrestrial culture. A beginning college student has had many experiences of communication success and failure across cultural and generation barriers, and it seemed like a good opportunity to make use of these experiences in exploring the possibilities and probabilities of communicating with ETI.
I volunteered to conduct a Freshman Seminar the fall of 1976 which would bear the presumptuous title "Communication with Extraterrestrial Intelligence." The course description handed to all incoming freshman students referred to attempts to answer questions like: "Why do we communicate?," "What do we communicate?," "When should we not communicate?," "How, other than verbally, can we communicate?." Limited to 15 students, the section soon filled and I felt somewhat put-upon to have to conduct the class for the first time with 19 students. I learned much that first year; mostly how inexperienced I was at exploring philosophical issues, keeping ahead of interested, curious students and most of all, how unprepared I was to stimulate good writers and good speakers to become even better.
Those of us who have been through the process have discovered that ten weeks is too short to do all we would like. With weekly written assignments and daily discussion sessions to prepare for, time runs out far quicker than ideas and questions.
I have worked traditional assignments into appropriate ETI formats. An introductory biography is prepared by each student (and the instructor) on a 20 by 30 element grid. The purpose is to have each student place as much information about himself as possible using a maximum of 600 characters, i.e. letters, digits or symbols. The objective is to transmit the maximum of ideas in the limited format. Class averages run about 20 ideas. Obvious limitations are set by traditions of writing, grammar, and lack of awareness of common, non-verbal modes of communication. Full meaning to the experience does not arrive until the "scientific picture" method of Frank Drake begins to pour idea upon idea later in the course.
In addition to the above exercises, an experience extending over the entire term is a class project to evolve our own estimates for values of each of the seven factors in the Drake equation for the estimated number of communicating technological civilizations in our galaxy (See COSMIC SEARCH, vol. 1, no. 1, Jan. 1979, page 34). A system of committee assignments puts each class member on two committees each charged with justifying an assumed value for one of the factors that goes into the calculation. With the value selected, each committee must arrive at limits on their uncertainty by specifying a maximum value and a minimum value for their variable along with a rationale for their choice. In spite of the fact that this is one of the most stimulating aspects of the course, college freshmen seem unable to arrive at and defend a thought of their own, even if they obtain help from other sources. Our educational system seems to turn them away from independence and creativity of thought.
For textbook reading I have used quite successfully, "ETI: the First Encounter" edited by James Christian, "Cosmic Connection" by Carl Sagan and "The Selfish Gene" by Richard Dawkins. Many other sources are used, some of which I list in the bibliography. Students respond very well to the Boston University Seminar, published by NASA in book form under the title "Life Beyond the Earth and the Mind of Man." It is very appropriate because we use the NASA film "Who's Out There" which includes segments of that seminar.
While I was observing at Kitt Peak National Observatory one of the visitors touring the McMath Solar Telescope introduced himself to me. When he found out I was teaching a course related to ETI he volunteered to send me reprints of some of his articles on the subject. As a result, part of our courses now include consideration of the idea of diplomatic exclusion of communication with ETI presented by Michael Michaud. (See COSMIC SEARCH, vol. 1, no. 3, Summer 1979, page 11). It turns out this is not an unpopular position for many students contemplating ETI for the first time.
The inclusion of original artwork depicting possible intelligent life forms proves to be an eyeopener for us all. Imagination, evolutionary awareness and a keen sensitivity to the value of non-violence in intellectual pursuits, if not in evolution, becomes evident as the group shares their beliefs and fears in drawings of alien beings.
Short stories based upon their view of ETI also reveal their awareness and apprehensions about the discovery that we are probably not unique in the universe. It may be of interest to note that only four of the 52 students who have taken this course were previous readers of science fiction. Practically all have been students without background in science. I am confident some have turned to look more favorably upon science as a result of their encounter with ETI.
As Carl Sagan points out, the searches and speculations about extraterrestrial intelligence certainly reveal to us far more about ourselves than it does about "them." I testify to the validity of his observation when college students investigate their role as stewards of intelligence awaiting the time when other intelligence becomes accessable to our minds.
Bibliography for a Course in Communication with Extraterrestrial Intelligence
Principal Resources:
Christian, James C. (Ed.) "ETI; the First Encounter" Prometheus Books, 1976
Sagan, Carl, "Cosmic Connection," Doubleday, 1973.
Berendzen, Richard, Ed. "Life Beyond the Earth and the Mind of Man" NASA, Scientific and Technical Information Office, Washington, D.C. 1973.
Supplemental Readings:
Sagan, Carl and Shklovski, I.S., "Intelligent Life in the Universe"
Ponnamperuma, Cyril and Cameron, A.G.W. "Interstellar Communication: Scientific Perspectives." Houghton-Mifflin, 1974.
Gunn, James E., "The Listeners." (A novel) Signet Books 1972
Sagan, Carl, "Dragons of Eden."
Dawkins, Richard, "The Selfish Gene," Oxford University Press 1976.
Bracewell, Ronald, "The Galactic Club: Intelligent Life in Outer Space," Freeman Press, 1974.
Magazines:
COSMIC SEARCH
Astronomy Magazine
Philip E. Barnhart is a member of the faculty of the Physics and Astronomy Department of Otterbein College (Westerville, Ohio). Dr. Barnhart received his Ph.D. degree from the Ohio State University.
|
The SEnTlnel
By: Robert S. Dixon
Project Oasis
A Progress Report
In the summer of 1979, NASA gathered together some 25 scientists to seek a method of sifting through
all the data that will be produced by the super-powerful SETI receivers now being designed and tested by NASA. The study program was held at the NASA-Ames Research Center near San Francisco, California, and lasted for 10 weeks. Many different methods were investigated and reported on in detail, resulting in a mountain of paper. The editing process continued throughout 1979 and 1980, aided by a reunion of some of the same scientists in the summer of 1980 at NASA-Ames. Eventually, a
condensed final report will be published for distribution, either by NASA or by the University of Santa Clara, who co-hosted the study. In addition, we plan to have a full-scale article on Project Oasis in COSMIC SEARCH, but until then, this report will serve to summarize the basic findings.
NASA is currently designing SETI receivers that have 10 million separate narrow-band channels. Each of these channels provides amplitude and phase measurements from two independent antenna polarizations once per second, for a total data rate of 40 million measurements per second. This rate is so large that the scientists coined a new unit of measurement to deal with it — Encyclopedias Per Second. Most normal computers cannot even record data this fast, much less process it in the very complex ways required to search for intelligent signals that may lie hidden in the data.
The greatest difficulty confronting the Oasis scientists was that of not knowing exactly what to look for. If you know beforehand what kind of signal will be received, it is easy to construct a receiver that will be optimized for that particular kind of signal. Since no one can be sure what kind of signals might be received from other civilizations, the problem is very difficult. The problem is more philosophical than technological in some respects, analogous to trying to find a single wind-blown footprint in a large lumpy desert.
A common technique used by scientists to analyze their data is called a transform. This is a mathematical operation that rearranges the data in such a way that the desired part of the data (the signal) becomes clearly separated from the rest of the data (the noise). There are many different kinds of transforms, and each kind is best suited to a certain class of problems. For example, Fourier transforms are used to analyze problems that involve sinusoidal waves, and Walsh transforms are used to analyze problems that involve square waves. One of the goals of the Oasis scientists was to find what they dubbed a "cartoon" transform, that would distinguish between any signal and noise. This goal was not realized, although it suggests areas for future research.
Having been unsuccessful in finding a single, elegant, all-powerful method for analyzing the SETI data, the scientists instead designed a three-pronged approach to the problem. One prong is an optimized detector for that kind of signal that most believe is the likeliest — a narrow-band carrier. This detector not only uses the narrowbandedness of the signal, but its polarization and time coherent properties as well. In addition, it allows for a range of possible doppler frequency drift rates.
The second prong is optimized for signals that are in a sense opposite to those of the first prong. It searches for pulsed signals, over a wide range of pulse rates.
The third prong is conceptually the most complex. It is a "shotgun" approach, using a variety of techniques that are not specific to any particular signal. The received data is grouped into blocks representing 40 hertz of bandwidth and 20 seconds of time. Each block is then characterized by its response to a group of tests. The tests include ANOVA (an analysis of the statistical properties of the block), coherence, polarization, correlation and probability distribution measurements. After each block is so characterized, then a second stage processer searches for patterns of similar characterization among a large number of neighboring blocks. In this way, signals that are strange and exotic could be detected without knowing their characteristics beforehand.
Behind the scenes in all these methods is acutally a fourth prong — a human being. The scientists felt that the information processing power of the human brain is very significant, and should be given every opportunity to augment the automated tests performed by the computer. Therefore the Oasis receiver design incorporates means of displaying the received and processed data to a trained operator (via visual and audible displays). The operator is thereby able not only to monitor the proper operation of the automated equipment, but to actively participate in the search for intelligent signals.
The summer study program also produced some unanticipated side benefits, one of which is the "SETI Song," presented here. It was first sung at the final banquet of Project Oasis, and undoubtedly is destined to inspire all future SETI workers down through the ages.
THE SETI SONG
By Norman Rhodine
University of Wyoming
(Sung to the tune of "Down by the Riverside")
They said the E.T.I.'s should be
Down by the Water Hole,
Down by the Water Hole,
Down by the Water Hole,
They said the E.T.I.'s should be
Down by the Water Hole,
Down by the Water Hole.
The following are sung as the first and fifth lines of each of the remaining stanzas with "Down by the Water Hole" as the 2nd, 3rd, 4th, 6th and 7th lines in the same manner as for the first stanza:
So we searched to find our distant friends
They attempted to communicate
At first their signal was too weak
We found our long sought E.T.I.'s
Then we asked our new friends "How are you?"
We waited such a long, long time
That when we got an answer back
We'd forgotten what the question was
We seemed to be light-years apart
So we decided that there was no hope
Say! What was that that I just heard
Just before you shut the receiver off
So we'll keep on searching without end
|
|
ABCs of Space
By: John Kraus
THE UNIVERSE IN SEVEN STEPS
The topic for this issue is a continuation of "The Universe in Seven Steps" taken from my new book OUR COSMIC UNIVERSE (Cygnus-Quasar Books).* (*Copyright© John D. Kraus 1980)
Step 1: "The Earth and Its Rings" was described in ABCs of Space in the Summer 1980 issue (Serial no. 7). Step 2: "The Earth-Moon System," Step 3: "The Solar System," and Step 4: "The Solar Neighborhood" were described in the last issue (Year End 1980, Serial no. 8).
In this issue we complete our journey across the universe via Step 5: "Our Galaxy," Step 6: "The Galactic Neighborhood" and Step 7: "The Universe."
For most of us, it is natural to think of our dwelling in relation to the yard outside, the local neighborhood, the town, the neighboring towns, the state or province, the country, the continent and the world.
In the same way, with a more cosmic perspective, all dwellers of Planet Earth should think of our home (the Earth) in relation to the Solar System, the Solar Neighborhood, our Galaxy, the Galactic Neighborhood and the Universe.
I hope that "The Universe in Seven Steps" helps to convey this perspective of our true place in the universe.
Step 5. Our Galaxy. The sun and 100 billion other stars form a huge aggregation called our galaxy (or Milky Way system), a flattened disc 100,000 light years in diameter turning like a great wheel once in 300 million years. The sketches show it as seen broadside and edge-on. In the nucleus at its center and in the long trailing spiral arms the stars are closer together than between the arms.
Surrounding the disc, like a swarm of bees, are a few hundred star clusters and a sprinkling of individual stars. These clusters and stars form a galactic halo, a spherical region centered on the nucleus. Some of the clusters may contain as many as 100,000 stars or more.
The solar system where we live is in a spiral arm about two-thirds of the way to the edge (30,000 light-years from the center). Most of the 500 brightest stars in the sky are relatively close (within 1000 light-years). The Crab pulsar and Cassiopeia A (Cas A) are further from the solar neighborhood.
The solar neighborhood sketch must be reduced by a factor of 5000 from the Step 4 diagram to be the correct size on the galaxy diagram. The dot showing the solar neighborhood should be about 100 times smaller in diameter to indicate its proper relative size. The sketch is idealized.
Step 6. The Galactic Neighborhood. Our galaxy and some nearby galaxies such as the Magellanic clouds and the Andromeda galaxy form the galactic neighborhood.
Our galaxy sketch must be reduced by a factor of 40 from the Step 5 diagram to be the correct size on the galactic neighborhood diagram. Our galaxy and the other galaxies are approximately the proper relative size.
Step 7. The Universe. The known universe contains our galaxy and 100 billion other galaxies out to a celestial horizon or observable limit at a radius of 15 billion light years. The other galaxies seem to be moving away as though the universe were expanding, the recession velocity increasing with the distance.
The galactic neighborhood is shown at the center with a few radio galaxies such as Cygnus A and 3C295 at increasing distances. The most distant known object, quasar OQ172, is receding at 91 percent of the velocity of light.
The galactic neighborhood diagram must be reduced by a factor of 7500 from the Step 6 diagram to be the proper relative size. The dots showing the galactic neighborhood and other objects are relatively much too large.
The light and radio waves we receive from the quasar O0172 have been traveling nearly 14 billion years to reach us. This means that we observe it, not as it is today, but as it was nearly 14 billion years ago. The farther an object is from us, the farther back in time we see it.
|
People
By: John Kraus
Philip Morrison, Institute Professor and Professor of Physics, Massachusetts Institute of Technology, is the recipient of the New York Academy of Sciences' Presidential Award. It was presented at the Academy's 163rd Annual Meeting held last December. Dr. Morrison was presented a certificate of citation, a medal, and $1,500 in recognition of his outstanding accomplishments as a distinguished scientist and interpretor of the relationship of science to society. Dr. Morrison then addressed the Academy with a talk entitled: "The Two Dials: Heartbeat and Earthspin."
Dr. Morrison is renowned for his professional contributions to theoretical astrophysics, including work aimed at expanding supernovae cosmic x-rays, disturbed galaxies, quasars and other questions in cosmology.
In addition to his role as a distinguished scientist, Philip Morrison is a respected scholar-philosopher and interpretor of science and technology for the general public. He is a leading expert on and advocate of strategic nuclear arms control. From 1943 to 1946 Dr. Morrison was associated with the Manhattan Project, which was responsible for the development of the first atomic bomb. In later years he opposed the development of the hydrogen bomb and became an unremitting critic of the strategic nuclear arms race. Dr. Morrison was a founding member of the Federation of American Scientists, a distinguished organization dedicated to science and public affairs, and was chairman of the Federation from 1973 to 1976.
The author of more than 75 professional scientific articles and more than 60 articles on science education and science for the general reader, Dr. Morrison is the co-author of two physics texts. In 1960 Professor Morrison received the American Association for the Advancement of Science – Westinghouse Science Writing Prize.
In 1955 Dr. Morrison received the Pregel Prize of The New York Academy of Sciences, and in 1967 the Babson Prize of the Gravity Foundation. During 1968-1969 he presented the 139th series of Christmas lectures in science for children at the Royal Institute of London which were televised by the British Broadcasting Corporation. In 1976, on the occasion of his 60th birthday, Philip Morrison was honored with a special issue of Technology Review.
In 1959 Philip Morrison with Giuseppe Cocconi, both then of Cornell University, published the first realistic strategy for searching for extraterrestrial intelligence to appear in a scientific journal (NATURE, London). By perceptive, incisive reasoning, the authors developed the proposal that a search be made of some of the nearest stars for signals at or near the 21 centimeter wavelength of neutral hydrogen.
On the 20th anniversary of this classic publication, it was reproduced in the premier issue of COSMIC SEARCH (January, 1979). Following it in the same issue of COSMIC SEARCH, Dr. Morrison reflected on the NATURE article with "Twenty Years After..." In this sequel he linked the past to the future with some very sage advice noting that "Whether we are alone in the universe or not needs to rest on experimental search, not on a string of evolutionary inferences."
Dr. Morrison is a member of the Editorial Board of COSMIC SEARCH.
•
Carl Sagan is now working on his first novel, "Contact," about man's first encounter with extraterrestrial beings. On the basis of Sagan's 115 page outline for the novel, Simon and Schuster is paying $2 million for the rights to publish the book scheduled to appear late this year. Movie rights for "Contact" have been purchased by Polyram Pictures which reportedly is budgeting $40 million to produce the film by late 1983.
Sagan's 13-week TV series "Cosmos" had its first run last fall and is now in re-runs.
Dr. Sagan is a member of the Editorial Board of COSMIC SEARCH.
•
A Public Contribution of $60,000 from 10,000 individuals was made to NASA in Washington on January 7 of this year in order that there be funds to continue operating the Viking lander on Mars for a couple more months. Organized by a group perturbed about cuts in the NASA budget, the gift demonstrates public concern that the U.S. not fall behind in the exploration of space. With the cooperation of the American Astronautical Society, the group believes that keeping the Viking lander in operation and sending back data on conditions on our neighboring planet is extremely important to our long range space program.
•
George E. Mueller, Deputy Administrator of NASA for Manned Space Flight during the Apollo missions to the moon, feels that the future of our space program depends in large measure on how well the Space Shuttle succeeds. He says that we are at a turning point where success could revitalize our program but continuing problems could set us way back.
Dr. Mueller points out that cuts in NASA's budget have forced it to concentrate on merely keeping a few existing programs alive with no provision for the long-range planning and development required for expanding or even sustaining our activities in space.
Widely recognized as the father of the Space Shuttle, Mueller believes that mankind's future lies in space. Dr. Mueller is President and Chairman of the Board of System Development Corporation of Santa Monica, California, and a recent past President of the American Institute of Aeronautics and Astronautics. Prior to his association with NASA Dr. Mueller was Professor of Electrical Engineering at the Ohio State University.
•
The Carter administration on January 18, 1981, called for a major, sustained national and international effort to cope with what it said were "increasingly critical global resource, environmental and population problems," according to the New York Times.
A report prepared jointly for the President by the State Department and the Council on Environmental Quality warned that excessive world population growth, dwindling resources and environmental degredation represent serious threats to the political and economic security of the United States.
The 250-page report contains recommendations for action by the United States, in concert with other nations, including a doubling of international resources devoted to family planning, greatly increased food aid, global plans to reverse the depletion of world forests and the spreading of deserts, and the conservation of critical resources such as energy and water.
The new report is a sequel to the "Global 2000 Report to the President," which was issued last summer and warned that, without action to reverse them, current trends would lead "to a more crowded, more polluted, less stable world" by the beginning of the next century.
The suggestions in the report have been offered for consideration and review by the Reagan administration, the Congress and the public. It was pointed out that these world problems affect everyone and transcend party politics.
•
A Manned Mission to Mars workshop is to be held at the University of Colorado April 29 - May 2, 1981, in order to plan the steps required for such a mission. For more information contact Chris McKay, Dept. of AstroGeophysics, Campus Box 391, Univ. of Colorado, Boulder, CO 80309. Tel. 303-444-0861.
•
The 92nd Scientific Meeting of the Astronomical Society of the Pacific will be held July 18 to 23, 1981, at the University of Washington, in Seattle.
The program includes: a Symposium entitled "Beyond the Main Sequence: Red Giants, Degenerate Stars, Black Holes, etc.," an Educator's Workshop on Astronomy in the Classroom, a non-technical lecture series on recent developments in astronomy, and a session for amateurs sponsored by the Northwest Region of the Astronomical League.
For more information send a stamped, self-addressed envelope to: Summer Meeting, A.S.P., 1290 24th Avenue, San Francisco, CA 94122.
JK
|
In Search of Planets
The Extrasolar Planetary Foundation Report
By: George Gatewood
The EPF spent its first quarter year obtaining legal counsel, drawing up papers of incorporation, and applying for a tax exempt status. This aside we turned to an announcement of our formation and our purpose. The latter brought a tremendous response from the national news media including TV, radio and newspapers and resulted in our first small donation. This in hand we opened a checking account and we have become a Foundation.
Now comes a period of financial growth and planning. A time of setting goals and finding means to reach them. In what ways can we best encourage and assist the search for extrasolar planetary systems? What is the minimum amount required to have a significant effect? What specific campaigns should we undertake?
Our Board of Directors met several times during the first quarter and we found many possible answers to these questions. To some extent the answers depend upon the funding available to the Foundation. For example, rather little funding is required to assist an astronomer in the preparation of a grant proposal to a Federal agency. But if he is more likely to get that grant because of our help we have bought a lot for a few dollars. On the other hand, the Foundation could support the construction and operation of an observatory with the sole purpose of detecting and studying extrasolar planetary systems. The freedom from the usual red tape involved in such a venture would allow quicker construction and assure the long-term operation required for a system which would reveal so many of the basic aspects of our Universe.
We will touch more on this in the next issue. In the meantime, for further information, write Extrasolar Planetary Foundation, Observatory Station, Pittsburgh, Pennsylvania 15214. Your tax deductible patronage will be greatly appreciated.
George Gatewood, Chairman
Extrasolar Planetary Foundation
|
Miscellaneous Items
Webpage Table of Contents (Bookmarks) (Internal links to categories of items in this webpage)
Information About the Publication (Editorial Board, Editors, Table of Contents)
Editors, and Others Involved in the Publication
Editor: John Kraus, Director, Ohio State University Radio Observatory.
Co-Editor: Robert S. Dixon, Assistant Director, Ohio State University Radio Observatory
Assistants: Alice Kraus, Jim Arthur
Editorial Board
- Richard Berendzen, President, The American University
- John Billingham, Director SETI Program, NASA-AMES Research Center
- Ronald Bracewell, Director, Radio Astronomy Observatory, Stanford University
- Thomas A. Clark, NASA-Goddard Space Flight Center
- Arthur C. Clarke, Sri Lanka, author of "2001, A Space Odyssey"
- Norman Cousins, Chairman, Editorial Board, SATURDAY REVIEW
- Frank D. Drake, Director, National Astronomy and Ionosphere Center (Arecibo), Cornell University
- Robert E. Edelson, SETI Project Manager, Jet Propulsion Laboratory, California Institute of Technology
- Donald S. Hall, Director, Strasenburgh Planetarium, Rochester, New York; Past President, International Planetarium Society
- Theodore M. Hesburgh, President, University of Notre Dame
- Nikolai Kardashev, Space Research Institute, Academy of Sciences, Moscow, USSR
- Philip Morrison, Physics Department, Massachusetts Institute of Technology
- Bernard Oliver, Vice President, Hewlett-Packard Company; Director of NASA-Ames Cyclops Project
- Cyril Ponnamperuma, Director, Laboratory of Chemical Evolution, University of Maryland
- Martin Rees, Director, Institute of Astronomy, Cambridge University, England
- Carl Sagan, Director, Laboratory for Planetary Studies, Cornell University
- Walter Sullivan, Science Editor, New York Times
- Vasevolod R. Troitsky, Radiophysical Scientific Research Institute, Gorky, USSR
- Sebastian von Hoerner, National Radio Astronomy Observatory
About COSMIC SEARCH
COSMIC SEARCH is published quarterly (Winter, Spring, Summer, Fall) by Cosmic-Quest, Inc. Copyright © 1980 by Cosmic-Quest, Inc. All rights reserved. Cosmic Quest, Inc., is a non-profit educational-scientific (tax-exempt) organization.
Subscription price: $10 for 4 issues in U.S. (and possessions), $13 elsewhere. Single copies: $2.50 in U.S. (and possessions), $3 elsewhere.
Address subscriptions and all other correspondence to: Radio Observatory, Box 293, Delaware, Ohio 43015.
Second-class postage is paid at Delaware, Ohio, and at additional mailing offices.
Opinions expressed by persons writing in COSMIC SEARCH are their own and do not necessarily reflect the opinions of the editorial staff.
Front Cover
The Orion nebula, birthplace of stars. The nebula visible in this photograph is several light-years across and is part of a much larger gas and dust region containing many different kinds of chemical elements and molecules. (U.S. Navy photograph).
Table of Contents (in magazine)
Item | Pg |
SETI and the Spectral Classification of Stars by John Bahng | 2 |
Not as We Know It by Isaac Asimov | 5 |
In Which Klingons Became Chimeras by Frank Drake
| 9 |
Universal Chemical Evolution by Robert Rubin | 10 |
ETI in the Classroom by Philip Barnhart | 16 |
Features | |
Editorial | 1 |
Letters | 14 |
College Courses | 15 |
The SEnTinel | 19 |
ABCs of Space | 20 |
People | 22 |
Back Issues (Subscription and order blank) | 23 |
In Search of Planets | 24 |
Photo Credits:
Annie J. Cannon (Shapley, Harlow, "Through Rugged Ways to the Stars," N.Y.: Charles Scribner's s Sons, 1969). Enjar Hertzsprung. Photo by D.Y. Martynov taken at 1958 Moscow meeting of the International Astronomical Union (American Institute of Physics, Niels Bohr Library, Shapley Collection). Henry Norris Russell (American Institute of Physics Niels Bohr Library,
W.F. Meggers Collection). William W. Morgan (Yerkes Observatory). Philip C. Keenan (Photo by Walter Mitchell). Representative spectra of stars in the principal spectral types (Courtesy of Perkins Observatory and Charles E. Merrill Publishing Company).
From the Editor's Kitchen Table
Continuation of the magazine, as explained in the last issue, is contingent on our holding costs below income and this required us to return to an all-volunteer staff. Because of the small staff and the need to wait until there was money on hand to pay the printing and associated costs, we fell behind schedule.
The last issue of COSMIC SEARCH (serial no. 8) was four months late, not being mailed by our printer at Fort Worth, Texas, until January 12. I expect that this issue (serial no. 9) will be about two months late but I hope that the Spring (April-May-June) 1981 issue (serial no. 10) can be on-time. Since distribution to all the contiguous 48 states by the U.S. Postal Service requires at least three weeks, this means that the issue will have to be mailed by the second week of March so as to reach most of the subscribers in the contiguous 48 states before April 1.
Of course delivery to more distant subscribers will require even more time (COSMIC SEARCH has subscribers in over 60 countries).
As a further economy, the serial no. 8 issue was reduced to 20 pages plus four page cover but more material per page was squeezed in by using smaller type and reducing margins. This issue is 24 pages plus four page cover. With subsequent issues I anticipate that it may be possible to further increase the number of pages. However, any increase in pages or a return to schedule is contingent on sufficient income from new subscriptions, renewals and contributions.
To keep the magazine going you can help by:
(1) renewing your subscriptions promptly
(2) making gift subscriptions
(3) telling others about COSMIC SEARCH and urging them to subscribe and
(4) making a gift or donation.
John Kraus
Thank You!
COSMIC SEARCH expresses sincere thanks to the following donors who are helping to make sure that the story of SETI and mankind's future continue to be told in an interesting and factual way.
Planetary Donors
Robert J. Allen, Blythdale, Missouri (2 years)
Jane L. Brooks, Adelaide, S. Australia (2 years)
Amahl S. Drake, Ithica, New York
James C. Killman, Sherman, Texas
David M. Laida, Sierra Vista, Arizona
Arthur J. Morgan, New York, N. Y.
A. V. Shaver, Winchester, Virginia
Paul Simons, Sheboygan, Wis.
Eric W. Six, Iowa City, Iowa
John B. Theiss, Tucson, Arizona
Dennis Wildfogel, Pomona, N.J.
One anonymous
Stellar Donors
George L. Douglass, II, Reno, Nevada
David C. Halley, Colonial Hts., Virginia
Paul Simons, Sheboygan, Wis.
John Teetor, Marion, Ohio
C.L. Turnage, Camarillo, California
Cosmic Donors
Two anonymous
COSMIC SEARCH, published by a non-profit scientific-educational organization, has been granted tax-exempt status by the Internal Revenue Service, so contributions are tax-deductible (but subscriptions are not). On a combination donation-subscription or donation-renewal, the amount over and above the magazine cost is tax deductible. For example, if a donor sends $30 (qualifying as a "Planetary Donor") and includes a two-year subscription at $18, the $12 difference is tax-deductible.
Donation categories are as follows:
Planetary: $30 per year
Stellar: $100 per year
Galactic: $500 per year
Cosmic: $1000 per year
Contributions, however, will be gratefully accepted in any amount. Checks should be made payable to Cosmic Quest, Inc., P.O. Box 293, Delaware, Ohio 43015.
COSMIC SEARCH GLOSSARY
- Anthropocentric:
- Man-centered.
- Astronomical Unit:
- A unit of length equal to the distance of the earth from the sun, about 150 million kilometers.
- Hertz:
- A unit of frequency equal to one cycle per second.
- Hydrogen:
- The most abundant element in the universe. It radiates naturally at a wavelength of 21 centimeters.
- Kelvin degrees:
- Absolute temperature measured in the celsius scale. Ten degrees kelvin equals ten degrees celsius above absolute zero.
- Light-Year:
- The distance traveled by light in one year, about 10 trillion kilometers.
- Macrocosm:
- The entire universe
- Microcosm:
- The universe in miniature
- Redshift:
- A shift toward the longer wavelengths of the optical spectrum due to recessional velocity (Doppler effect).
- SETI:
- An acronym for Search for Extra-Terrestrial Intelligence.
- Universe:
- The ultimate structure which contains us, our galaxy and all other galaxies.
Miscellaneous Quotes
The following quotes are not directly associated with any article.
Quotes on inside back cover
Ozma Revisited (with apologies to Dr. Doolittle)
If we could talk to the Aliens
Just imagine it
Gabbing all throughout the Galaxy;
Imagine chatting with a creature
Who lives near Tau Ceti
What a neat achievement that would be.
If we communed through the Universe
Via ultra-cee
Think how fantastic it would seem;
We might postulate a pulsar
Broadcasting beyond light-speed,
Or modulate a tight tachyon beam.
We might converse
By 21-centimeter wavelength
Exchanging verse
With chaps light-years away;
And if they ask, "Can you
Speak telepathically?"
We'd say emphatically,
"Some day!"
If we could talk to the Aliens,
Learn their languages,
Maybe take a course to learn ETese;
We'd study languages celestial
From profs extra-terrestrial
On radio beamed here from Antares.
We'd study every living creature's language,
And even some
Of whom we're not so sure;
And if asked, "Do you
Speak veg and mineral?"
We'd say "Indeed, we will,
If they concur."
If we consulted with multipeds
Think of all we'd learn
Inquiring about their society;
We'd hear homilies from hive-minds;
And allegories, all kinds,
From flora and fauna microscopically.
If we sent codes to crustaceans
The advantages
Any one on earth could plainly scan,
Exchanging platitudes with plasmas
And sentient miasmas
That's a big step forward for all Man.
And we are sure every sentient,
Man and Alien,
Certainly would sense it as a plus
If we could talk to the Aliens
Speak to the Aliens,
Esp or psi or grok with the Aliens
And they would talk to us!
The Year 3000 Looks Back at the Launching of the Space Shuttle
Once humans thought their home was Earth,
And everything beyond those walls
Of familiar blue was foreign;
But someone opened a door
And stepped outside, and now
Our house is larger than at first:
Now we call our home the universe.
THE BALLAD OF OQ172
Way out in space further than you can ponder,
Where the cosmos ends and there is no yonder,
Is a quasar so strong and bright
It bends your mind right out-of-sight.
Beginning eons ere the earth was born,
In a cosmos void and all forlorn,
Came a horrendous blast of fire and flame
And thereafter the heavens were never the same.
Now the quasar was a white-hot ball of fire
Flying at light-speed across a vast empire,
Spinning around like a spaced-out dervish
And sending out waves both straight and curvish.
It's bigger than a billion suns,
It's very old but it runs and runs,
It's speeding faster than a million jets,
And it's size even tops all the U.S. debts.
It's a quasar of great fame,
O-Q-1-7-2 is its name.
The more we learn,
The more we learn,
How little we learn.
I WONDER
Why would so great a firmament
Create itself for us
And move its solar systems out
In perfect harmony
To monitor both time and space
Of relative pursuit
A carnival of moons and stars
That we alone enjoy?
Some alien intellects must know
Dimensions we cannot
Yet comprehend in terms like theirs
To learn which paths to plot.
Our time will come, it doubtless must
An Einstein guarantee
If E but equals MC2
In heaven's astronomy.
|
|